Pitfalls in lung stereotactic body radiotherapy—a review of organ toxicities and dose constraints
Introduction
Stereotactic body radiotherapy (SBRT), which is also known as stereotactic ablative radiotherapy (SABR), has been established as a treatment for early-stage inoperable non-small cell lung cancer (NSCLC) in several international guidelines. One of the earliest phase I study data in lung SBRT was first published in 2003 by Timmerman and colleagues (1), with the same group subsequently reporting excellent phase II results (2). These two trials and similar landmark studies, now form the basis for lung SBRT practice internationally.
Although SBRT is a proven treatment option for early stage inoperable NSCLC, it is still fairly new and not available in many developing parts of the world. There are still uncertainties with regards to optimal radiotherapy dose-fractionation regimen and because of the large ablative doses used in SBRT, there is a potential for significant side effects. In this paper, we elaborate on the common toxicities reported in literature in relation to SBRT of lung tumors as well as factors that may increase these risks.
Organs at risks (OAR)
The common toxicities reported in literature relating to SBRT of lung tumors can be divided into general radiation therapy related, like fatigue, and specific toxicities relating to structures within the thorax such as the heart, chest wall (CW), lung, major airways, greater vessels and esophagus. Collectively, these structures are known as organs at risks. This is especially important to tumors located close to these structures [e.g., centrally located tumors (Figure 1)] or in situations where a patient may be at greater risk of toxicities (e.g., patients with reduced lung function).
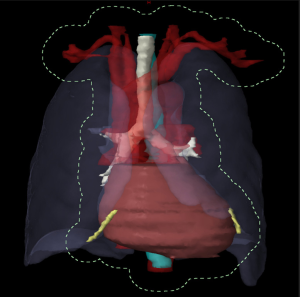
The radiobiological effects of extreme hypofractionation on tissues are not fully understood (5-7) and existing models may not provide accurate normal organ tolerances (8). It is thus not advisable to freely apply the OAR dose limits used in conventional radiotherapy on SBRT. However, due to the lack of long-term data and limited patient numbers, the linear-quadratic model is still often used for radiotherapy planning.
In 2010, with the increasing use of SBRT, the American Association of Physicist in Medicine Task Group 101 (AAPM TG101) (9) published their recommendations of OAR dose constraints (Table 1) in the first attempt at guiding radiotherapy planning. Notably, most of the recommended constraints were derived anecdotally and not validated. Subsequent published studies have helped refine organ constraints further with reasonable toxicity rates reported so far.
Table 1
Guideline | AAPM TG 101 (9) | RTOG 0236/0618 (2,10) | RTOG 0915 (11) | RTOG 0813 (12) | JCOG 0702 (13) | UK SABR consensus (14) | ||||||||||||||||
---|---|---|---|---|---|---|---|---|---|---|---|---|---|---|---|---|---|---|---|---|---|---|
Vol (cc) | DC (Gy) | Vol (cc) | DC (Gy) | Vol (cc) | DC (Gy) | Vol (cc) | DC (Gy) | Vol (cc) | DC (Gy) | Vol (cc) | DC (Gy) | |||||||||||
1# | 3# | 5# | 3# | 4# | 5# | 4# | 1# | 3# | 5# | 8# | ||||||||||||
Spinal cord+ | Max | 14 | 21.3 | 30 | Max | 18 | Max | 26.0 | Max | 30 | Max | 25 | Max | 10ˆ/14* | 18ˆ/21.9* | 23ˆ/30* | 25ˆ/32* | |||||
<0.35 | 10 | 18 | 23 | – | – | <0.35 | 20.8 | <0.25 | 22.5 | – | – | 1 | 7ˆ | 12.3ˆ | 14.5ˆ | |||||||
<1.2 | 7 | 12.3 | – | – | <1.2 | 13.6 | <0.5 | 13.5 | – | – | – | – | – | – | – | |||||||
Brachial plexus | Max | 17.5 | 24 | 30.5 | Max | 24 | Max | 27.2 | Max | 32 | – | – | Max | – | 24ˆ/26* | 27ˆ/29* | 27ˆ/38* | |||||
<3 | 14 | 20.4 | 27 | – | – | <3 | 23.6 | <3 | 30 | – | – | – | – | – | – | – | ||||||
Lungs~ | 1,500 | 7 | 11.6 | 12.5 | V20 <10% | 1,500 | 11.6 | 1,500 cc | 12.5 | V20<37% | – | – | – | – | – | |||||||
1,000 | 7.4 | 12.4 | 13.5 | – | – | 1,000 | 12.4 | 1,000 cc | 13.5 | – | – | – | – | – | – | – | ||||||
Esophagus | Max | 15.4 | 25.2 | 35 | Max | 27 | Max | 27 | Max | 105% PD | ≤1 | 40 | – | – | – | – | – | |||||
<5 | 11.9 | 17.7 | 19.5 | – | – | <5 | 18.8 | <5 | 27.5 | ≤10 | 35 | – | – | – | – | – | ||||||
Heart/pericardium | Max | 22 | 30 | 38 | Max | 30 | Max | 34 | Max | 105% PD | – | – | Max | – | 24ˆ/29* | 27ˆ/29* | 50ˆ/60* | |||||
<15 | 16 | 24 | 32 | – | – | <15 | 28 | <15 | 32 | – | – | – | – | – | – | – | ||||||
Great vessels | Max | 37 | 45 | 53 | – | – | Max | 49 | Max | 105% PD | ≤1 | 40 | Max | – | 45* | 53* | – | |||||
<10 | 31 | 39 | 47 | – | – | <10 | 43 | <10 | 47 | ≤10 | 35 | – | – | – | – | – | ||||||
Major Airways | Max | 20.2 | 30 | 40 | Max | 30 | Max | 34.8 | Max | 105% PD | ≤10 | 40 | Max | 30ˆ/32* | 32ˆ/35* | 32ˆ/44* | ||||||
<4 | 10.5 | 15 | 16. | – | – | <4 | 15.6 | <4 | 18 | – | – | – | – | – | – | – | ||||||
Skin | Max | 26 | 33 | 39.5 | Max | 24 | – | – | Max | 32 | Max | 40 | Max | – | 33ˆ | 39.5ˆ | – | |||||
<10 | 23 | 30 | 26.5 | – | – | – | – | <10 | 30 | – | – | 10 | – | 30ˆ | 36.5ˆ | – | ||||||
Chest wall | – | – | – | – | – | – | – | – | – | – | – | – | Max | – | 37ˆ | 39ˆ | 39ˆ | |||||
– | – | – | – | – | – | – | – | – | – | – | – | 30 | – | 30ˆ | 32ˆ | 35ˆ | ||||||
Rib | Max | 30 | 36.9 | 43 | – | – | Max | 40 | – | – | – | – | – | – | – | – | – | |||||
<1 | 22 | 28.8 | 25 | – | – | <1 | 32 | – | – | – | – | – | – | – | – | – | ||||||
<30 | – | 30 | – | – | – | – | – | – | – | – | – | – | – | – | – | – |
#, fraction; ^, optimal dose constraint; *, maximum allowed dose constraint. Vol, threshold volume; DC, dose constraints; Max, maximum point dose to organ; PD, prescribed dose; +, spinal cord planning organ at risk (PRV) is used in some guidelines (PRV = OAR + 3–5 mm expansion). ~, lung OAR = Total Lung volume – Gross Tumour Volume (GTV); OAR, organ at risk.
Reported toxicities in prospective series
Many well conducted prospective trial protocols have published OAR dose limitations (Table 1). These serve as guidelines for many centers and allow the safe and effective delivery of SBRT techniques.
Radiotherapy related toxicities are graded by severity based on the Common Terminology Criteria for Acute Adverse Events (CTCAE) (15) or the RTOG/EORTC (16) late radiation morbidity scoring schema. However, a common problem when reporting toxicities is the interplay of other factors e.g., infection, systemic treatment toxicities or comorbidities like chronic obstructive pulmonary disease (COPD) and tumor progression that can make the direct attribution from radiotherapy alone hard to interpret. Nonetheless, grade 3 toxicities and above (≥ G3) are considered severe as there would be impairment of function requiring some form of intervention.
A literature review of SBRT primary lung prospective trials was performed. Nineteen trials, from 21 published articles and 5 abstracts were found, with a total of 1,381 patients reported. Data that was reported from the same center and trial were combined as the patients were assumed to be from the same cohort. The study, trial type, size, tumor criteria, radiotherapy dose-fractionation, median follow-up and reported ≥ G3 toxicities are tabulated (Table 2). Reported ≥ G3 toxicities rates ranged from 0–29.8%. There were 21 cases of grade 5 (G5) toxicities.
Table 2
Study | Trial type | Patient numbers | Criteria | Treatment dose (Gy/fraction) | Median follow-up (months) | Toxicity (graded 3 and above) | Remarks |
---|---|---|---|---|---|---|---|
Timmerman et al. 2006 (3), Fakiris et al. 2009 (17) (Indiana) | Ph 2 | 70 | Inoperable, any location, T≤7 cm | T1 60 Gy/3#; T2 66 Gy/3# | 50.2 | G3-4 AE (10%)—6 Pulmonary, 1 Anxiety. G5 AE (7.1%)—4 pulmonary, 1 hemoptysis | 5/48 peripheral vs. 6/22 central G3-5 events (P=0.088); FEV1 and DLCO did not predict for RT toxicities (18); DLCO decline 1.11 mL/min/mmHg/y (P<0.001) |
Baumann et al. 2009 (19) | Ph 2, multicenter European | 57 | Inoperable, peripheral, T≤5 cm | 45 Gy/3# | 35 | G3-4 AE (29.8%), 11 pulmonary, 3 CW, 1 fatigue, 1 heart | |
Timmerman et al. 2010, 2014 (RTOG 0236) (2,20) | Ph 2, multicenter North American | 55 | Inoperable, peripheral, T≤5 cm | 60 Gy/3# (54 Gy/3# with density heterogeneity) | 34.4 | G3 AE 15/55 (27.3%), G4 AE 2/55 (3.6%), G5 AE 0 | At 2 years FEV1 and DLCO declined 5.8% and 6.3% respectively. PFT and dosimetric Lung parameters did not correlated with risk of RP (21) |
Ricardi et al. 2010 (22) (Torino) | Ph 2 | 62 | Peripheral, T≤5 cm | 45 Gy/3# | 28 | G3 RP (3.2%). 1 rib fracture, 3 (4.8%) chronic pain syndrome | MLD main predictor of toxicity; no change in FEV, TLV, VC (23) |
Le et al. 2006 (24) (Stanford) | Ph 1, dose escalation | 32 | Inoperable, any location, T ≤5 cm, primary/recurrent/mets | 15 Gy single fraction at 5 Gy dose increments to 30 Gy | 18 | G3 pulmonary 1/32 (3.1%), G5 3/32 (9.4%) | All G5 had receive chemo, 2 had prior radiotherapy; most toxicities in central tumors, G3 happened at ≥25 Gy; no change in FEV1, FVC or DLCO at 3 months |
Fritz et al. 2006 (25) (Marburg) | Prospective | 58 | Inoperable, T ≤5 cm, primary/mets | 30 Gy/1# | 22 (mets); 18 (primary) | no cases of ≥G3 AE | |
Videtic et al. 2015 (11) (RTOG 0915) | Randomized | 84 | Inoperable, peripheral, T <5 cm | 34 vs. 48 Gy/4# | 30.2 | 34Gy/4# ≥G3 AE (10.3%), 1 G5 AE (not-RT related). 48 Gy/4#: ≥G3 AE (13.3%), 1 G5 AE (pulmonary) | |
Nagata et al. 2015 (26) (JCOG0403) | Ph 2 | 164 | Any location, T ≤3 cm | 48 Gy/4# | 67 | G3 AE (8.9%), G4 AE (1.2%); no G5 | |
Timmerman et al. 2013 (10) (RTOG 0618) | Ph 2 | 26 | Operable, peripheral, T ≤5 cm | 54 Gy/3# | 25 | G3 AE (15.4%) no G4-5 | |
Allibhai et al. 2013 (27) (PMH) | Prospective | 185 | Inoperable, any location, T1–2 | Peripheral: T1 48 Gy/4#, T2 54–60 Gy/3#; central: 60 Gy/8# or 50 Gy/10# | 15.2 | G3 RP (1.8%) no G4-5 RP | RP not associated with V20 Gy or MLD |
Timmerman et al. 2003 (1), McGarry et al. 2005 (28) (Indiana) | Ph 1, dose escalation | 47 | Inoperable, Any location, T <7 cm | 24 Gy/3# at 6 Gy/# dose increments to 72 Gy/3# | NR | G3-4 AE (14.9%)—4 pulmonary, 1 skin, 1 pericardial effusion, 1 trachea necrosis | |
Shibamoto et al. 2012 (29,30) (Nagoya) | Prospective, multicenter Japanese | 180 | Inoperable, any location, T ≤5 cm | T <1.5 cm: 44 Gy/4#; T 1.5–3 cm: 49 Gy/4#; T >3 cm: 52 Gy/4# | 52.5 | G3 RP (1.1%) and pleural effusion (0.6%), ≥ G2 esophagitis (1.7%), rib fracture (2.2%) and dermatitis (3.9%) | |
Taremi et al. 2012 (31) (PMH) | Prospective | 108 | Inoperable, any location, T1-2 | Peripheral: 48 Gy/4#, 54 Gy/3#, 60 Gy/3#; central: 60 Gy/8#, 50 Gy/10# | 19.1 | G3 early AE (3.7%), 2 pulmonary, 1 fatigue, 1 CW. G3 late AE (5.6%)—3 CW, 3 pulmonary; no G4-5 AE | |
Bezjak et al. 2016 (12,32) (RTOG 0813) | Ph 1/2, dose escalation | 120 | Inoperable, central, T <5 cm | 50 Gy/5# at 0.5 Gy dose/# increments to 60 Gy/5# | 26.6 | ≥ G3 AE (12.5%); 4 G5 AE | |
Chang et al. 2015 (33) | Pooled analysis, 2 RCTs STARS and ROSEL | 31 | Operable, any location (STARS), peripheral (ROSEL), T <4 cm | STARS: peripheral 54 Gy/3#, central 50 Gy/4#; ROSEL: 54 Gy/3# or 60 Gy/5# | 40.2 | G3 AE (10%)—dyspnea/cough, chest wall pain, fatigue; no G4-5 AE | |
Lindberg et al. 2017 (34) (Nordic-HILUS) | Ph 2, multicenter nordic | 74 | Inoperable, central, T <5 cm, mets or primary | 56 Gy/8# | NR | ≥G3 AE (28%); 7 G5 AE (6 hemoptysis, 1 pneumonitis) | Tumors close to main stem vs. lobar bronchus increased risk of G4-5 AE (19% vs. 3%) |
Kimura et al. 2017 (35) (JROSG10-1) | Ph 1, multicenter Japanese, dose escalation | 10 | Inoperable, central, T ≤3 cm | 52–60 Gy/10# | 39 | No cases of ≥ G3 AE | |
Onimaru et al. 2015, 2017 (13,36) (JCOG 0702) | Ph 1, multicenter Japanese, dose escalation | 28 | Peripheral, T =3–5 cm, PTV <100 cc | 40 Gy/4# at 5 Gy total dose increments to 60 Gy/4# | 28.8 | No cases of ≥ G3 AE | |
13 | Peripheral, T =3–5 cm, PTV ≥100 cc | 40 Gy/4# at 5 Gy total dose increments to 50 Gy/4# | 45.5 | No cases of ≥ G3 AE | |||
Nagata et al. 2005 (37) (Kyoto) | Ph 1/2 | 45 | Any location, T <4 cm | 48 Gy/4# | 30 | No cases of ≥ G3 AE | |
Bral et al. 2011 (38) (Belgium) | Ph 2 | 40 | Any location, T ≤6 cm | Peripheral: 60 Gy/3#; central: 60 Gy/4# | 40 | ≥G3 AE (20%)—All pulmonary; no G5 AE |
Ph, phase; mets, metastastic lesion; T, tumour size/stage; AE, adverse events; #, fraction; G, grade; CW, chest wall; FEV1, forced expiratory volume in 1 second; PFT, pulmonary function test; DLCO, diffusing capacity of the lungs for carbon monoxide; FVC, forced vital capacity; RP, radiation pneumonitis; MLD, mean lung dose; V20 Gy, volume of lung receiving 20 Gy.
From Table 2 we see that SBRT doses vary between centers. Choice of dose regimen and are often determined by physician comfort, tumor and patient characteristics. These trials along with other retrospective series have increased our appreciation for the toxicities in lung SBRT and provided the evidence for organ dose tolerances. Recognizing these potential toxicities allows us provide an informed decision and allow for safe delivery of treatment.
Toxicities by site
Lung
One of the most common toxicities related to SBRT of the lung is radiation pneumonitis (RP) (Figure 2), which can range from asymptomatic to symptomatic breathlessness, fevers, cough and even death from respiratory failure. The non-specific presentation of RP and contributory comorbidities can make it difficult to distinguish an acute episode of radiation induced pneumonitis from other differentials like pneumonia or COPD exacerbations. This makes the comparison of incidence between studies difficult.
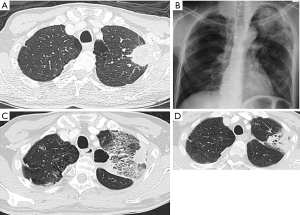
In a large pooled cohort study of 505 cases (39), the rate of ≥ G2 and ≥ G3 pneumonitis was 7% and 2% respectively. There was 1 case (0.2%) of G5 pneumonitis. Time from RT to onset of pneumonitis was a median of 0.4 years. The risk can vary with some series reporting up to 12% cases of life-threating RP (3,40). The risk of toxicities is noted to be more in patients with central and large lesions (3,41). Mid-lower lung lesions (42-44) are also at an increased risk of RP. This may partly be attributed to greater radiosensitivity of the area, functional importance of the lower lung (45) and increased respiratory motion (44), which results in a larger planning target volume (PTV).
An association between different dosimetric parameters and rates of RP has been noted in several studies. Barriger et al. reported mean lung dose (MLD) >4 Gy (4.3% vs. 17.6%; P=0.02) and a volume of lung receiving 20 Gy (V20 Gy) >4% (4.3% vs. 16.4%; P=0.03) being associated with an increased risk of G2-4 RP (46). Yamashita et al. and Inoue et al. similarly reported the predictive significance of MLD and V20 Gy (40,47). The ipsilateral MLD >9.14 Gy, total lung V5, V15, V20, V25 and V40 Gy ≥6.3% have also been associated with higher rates of RP (48-50). Doses to the contralateral lung V5 Gy >26% and MLD >3.6 Gy have been associated with increased rates of RP as well (51,52). Another parameter looked at is the conformity index (CI) which measures the ratio of volume treated to prescribed dose over the PTV. This is ideally kept as close to 1 as possible, to ensure the high dose region conforms to the target volume. Notably, RP occurs at a significantly increased frequency in patients with poor CI (P=0.0394) (40).
Patient related factors such as older age, pre-existing pulmonary comorbidities and female gender are associated with increased risk of RP (53-56). Interestingly, smoking was found to be protective against RP in some studies (54,57-60). Genetic factors such as single nucleotide polymorphisms (SNPs) of heat shock protein beta-1 (HSPB1) (61), methylene tetrahydrofolate reductase gene (MTHFR; rs1801133) (62), vascular endothelial growth factor (VEGF) gene (63), ataxia-telangiectasia mutated (ATM) (64-66) and Nijmegen breakage syndrome 1 (NBS1) genes (66) are also at increased risk of RP.
A pulmonary function test (PFT) done before SBRT does not always predict for RP (21,67). Changes in post-treatment PFT are usually minimal (18,23,24,68), although it will be decreased in the presence of RP or lung disease. The presence of baseline interstitial lung disease (48,49,69-71) and collagen vascular disease (CVD) (72) is a significant predictor of RP. In patients with idiopathic pulmonary fibrosis, the pre-treatment 18F-fluorodeoxyglucose (FDG) on positron emission tomography (PET) non-target lung uptake may serve as a biomarker for degree of baseline inflammation and predict the risk for RP (73).
Inflammatory cytokines to predict for RP has been extensively studied. Plasma transforming growth factor beta (TGFβ) is a cytokine which stimulates proliferation of fibroblasts, collagen synthesis and associated pulmonary fibrosis. It is chronically elevated in patients with CVD (72). TGFβ-1 polymorphisms (74) and elevated levels of TGFβ-1 after radiotherapy have been associated with an increased RP risk (75,76). Other serum biomarkers such as elevated pre- & post-treatment interleukin (IL)-6 (77,78) and IL-8 (79) have been associated with higher rates of RP. Pre-treatment levels of Krebs von den Lungen (KL-6), a circulating mucin-like glycoprotein expressed and secreted from bronchial epithelial cells and type II pneumocytes, and lung surfactant protein (SP-D) are also associated with an increased risk of severe RP (80). Elevated post-treatment KL-6 levels are correlated to higher RP incidence as well (81).
To date, these markers are not routinely screened and have not been prospectively validated for clinical use. In the future, a combination of biomarkers, patient and dosimetric parameters may improve the ability to predict RP and allow for better individualization of target doses to reduce lung toxicities.
Heart
A wide spectrum of heart toxicities are noted following conventional radiotherapy. Early toxicities include pericarditis and pericardial effusion. Late toxicities manifest 10–15 years after radiotherapy as disease of the coronary arteries, heart valves, conductive system and myocardium. Microvascular changes and accelerated coronary sclerosis are thought to contribute to the process (82). Studies on radiation effects of the heart are mostly obtained from conventionally treated breast and mediastinal lymphoma patients. Darby et al. showed a 7.4% increased risk of ischemic heart disease per Gy (mean heart dose) (83). An RTOG 0617 trial randomized patients between high vs. standard conventional dose RT for lung cancer and reported heart V5 and V30 Gy as significant predictors for worst survival (84,85).
The effects SBRT doses have on the heart are less understood. A large retrospective analysis of 803 patients treated with SBRT reported that maximum point dose (Dmax) to the left atrium of median 6.5 Gy (P=0.035) and dose to 90% of the vena cava (D90%) of median 0.59 Gy (P=0.008) were both associated with non-cancer deaths (86). Increased cardiac uptake on FDG PET after SBRT may be a marker of radiation induced myocardial injury and was noted to be associated with a cardiac V20 Gy >5 cm3 (87).
An institution in Florence treated 16 patients with paracardiac/cardiac lesions up to 36 Gy in 3 fractions at the 70% isodose line. At a median follow-up of 6.7 months, no cardiac event or echocardiography changes were noted (88). However, it is important to note that SBRT patients tend to have a better prognosis and therefore a longer time to develop late heart toxicities. It is thus advisable to keep heart doses to a minimum during radiotherapy planning.
Major vessels
Major vessels include the aorta, pulmonary vessels and superior vena cava. Radiation damage to these structures can result in hemoptysis, exsanguination secondary to rupture, aortic aneurysms or dissection and pulmonary hemorrhage.
Xue et al. recently published a logistic dose-response model for aorta and major vessels based on a total of 625 cases. They estimated that at Dmax =52.5 Gy in 5 fractions, the risk of grade 3–5 toxicity was 1.2%. At Dmax =45 Gy in 3 fractions, the risk was 2.3% (89). They concluded that following internationally recommended constraints, sufficiently high doses can be achieved with low risks to the vessels.
Central airways
The proximity of centrally located lesions increases the risk of central airways toxicities. This can result in atelectasis, stenosis/stricture, airway necrosis, fistula formation or even fatal hemoptysis (90). A phase II study from Indiana University reported 11-fold higher risk of G3–5 toxicities, with 4 deaths associated with central location (3). The most common severe toxicity in the trial were hemoptysis, stenosis, airway occlusion and fistula formation. At median follow-up of 50.2 months, the risk of toxicity remained higher compared to peripheral lesions (27.3% vs. 10.4%) (17).
At conventional high dose (≥73.6 Gy) RT, Kelsey reported a 6% to 57% narrowing of mainstem bronchus in 17 of 18 patients (91). The degree of stenosis was dose dependent and progressed with increasing time after radiotherapy. It was worse if the patient received chemotherapy.
With SBRT, Song et al. reported that at doses of 40–60 Gy in 3–4 fractions to central tumors, 8 of 9 patients (89%) showed complete or partial bronchial stricture at a median follow-up time of 26.5 months (92). Karlsson et al. prescribed doses of 20–50 Gy in 2–5 fractions for central tumors and reported incidence of 24.3% radiation-induced atelectasis at a median time of 8 months (1.1–30.1 months). On analysis, there was a dose dependent correlation. The median 2 Gy equivalent doses to 0.1 cm3 of bronchial tree in patients who developed atelectasis was 210 vs. 105 Gy in who did not (P=0.031) (93).
Duijm et al. reported results in 134 patients treated with SBRT to central tumors. They correlated toxicities in different parts of the airway with dosimetric parameters. Higher grade toxicities such as occlusion and atelectasis were reported in the lobar and segmental bronchi. When 0.5 cc of segmental bronchi was irradiated to 50 Gy in 5 fractions, the likelihood of occlusion was 50%. For the mid- and mainstem bronchi, the 50% risk to develop grade 1 radiographically evident side effects was a Dmax of 55 and 65 Gy respectively (94).
Fatal hemoptysis attributed to high-dose RT are uncommon but have been reported in literature (95-98).
Esophagus
The esophagus is another dose limiting organ in central lung SBRT. Reported side effects range from mild esophagitis to life-threatening strictures, perforations and trachea-esophageal fistulas (1,99). Cox et al. reported 6.8% ≥G3 esophageal toxicities in 182 patients treated with 24 Gy single fraction paraspinal radiosurgery (100). The incidence increased with higher doses-volumes. At the median split D2.5 cm3 >14.02 Gy, the risk was increased 6-times (P=0.01). They recommended a Dmax of 22 Gy.
However, despite keeping SBRT doses-volume to constraints that are considered safe (D5 cc 14.5 Gy, D2 cc 15–20 Gy, and Dmax of 19 Gy), Abelson et al. reported 2 incidence of high grade toxicities (esophageal fistula and esophageal perforation) in 31 patients (101). Notably, these 2 patients had received chemotherapy which is felt to be a co-factor.
In a systematic review, which included data from 563 central lung tumors from 20 studies, the incidence of G3–4 toxicities was 8.6% (102). The risk of treatment related mortality was reduced when tumor biological equivalent dose (BED) was <210 Gy (3.6% vs. 1%). The authors concluded that acceptable control and limited toxicities could be achieved with appropriate fractionation regimens e.g., 50 Gy in 5, 54 Gy in 6, 56 Gy in 7 and 60 Gy in 8.
Further research is needed to establish more reliable dose limits for mediastinal structures. The RTOG 0813 (12,32), Nordic-HILUS (34) and EORTC LungTech (103) trials are phase II SBRT trials that will provide a better understanding of treatment of central lung tumors.
CW, skin and ribs
CW pain, rib fractures (Figure 3) and skin toxicities are associated with peripheral lung tumors. The mechanisms for CW pain are unclear and suspected to be due to damage of soft tissue, neurovascular bundle and bone (104). The correlation between rib fractures and CW pain is less certain, with majority of CW pain presenting without rib fractures or vice versa (105,106).
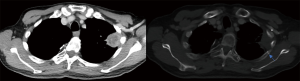
Creach et al. reported that CW doses receiving V30–40 Gy was most predictive of CW pain. A V30 Gy threshold of 0.7% and V40 Gy threshold of 0.19% was correlated with a 15% risk of CW pain (106). Mutter et al. reported similar findings in that V30 Gy >70 cm3 correlated with the presence of CW pain (104). Stephans et al. reported similar dose volume constraints and by maintaining V30 Gy ≤30 cm3 and V60 Gy ≤3 cm3 should result in a <10–15% risk of late CW toxicity (107).
Grills et al. reported in 483 patients, the incidence of rib fracture was 8% at median time of 0.9 years. This was associated with a higher BED and the optimal BED cut off was 132 Gy (11% vs. 5%; P=0.007) (39). Other reported parameters for dose constraints to rib or CW are Dmax =50 Gy, V40 Gy <5 cc (105) and the dose to 2 cm3 of rib (D2 cm3). At rib D2 cm3 <21 Gy/3#, the risk of the ribs fracture is close to 0%. At 27.3/3# and 49.8/3#, the risks are 5% and 50% respectively (108).
Obesity, diabetes and posterior tumors close to skin surface at an increased risk of skin and CW toxicities (109,110). Treatment factors to reduce skin toxicities should be undertaken by reducing the bolus effects from immobilization devices, calculating the skin doses and using multiple non-coplanar beams. The recommended constraints to skin reduce toxicities were reported as Dmax <50% prescribed dose (110), and reducing the volume of the CW receiving 30 Gy (V30 Gy <50 mL, 22% vs. ≥51 mL, 44%; P=0.02) (109).
Bonger’s et al. compared different fractionation regimens in which 60 Gy was delivered in 3, 5 or 8 fractions depending on tumor location and did not note a significant difference between CW toxicities (111). However, in challenging cases not meeting dose constraints, longer fractionation regimens e.g., 70 Gy in 10 fractions may be a suitable alternative to reduce the risk of CW toxicities and produce similar local control rates (112).
Brachial plexus
Brachial plexus injury can happen during SBRT of apical lung tumors. Injury to the plexus can present as neuropathic pain in the shoulder or arm, motor weakness or paresthesia (113). Chang et al. reported that in patients with central tumors, the brachial plexopathy incidence was higher when Dmax >35 Gy and V30>0.2 cm3 (P=0.001) (114). By limiting total dose delivered to the plexus to <26 Gy in 3–4 fractions, there would be a decreased risk of brachial plexopathy at 2 years (46% vs. 8%, P=0.04) (115).
Thoracic re-irradiation
Recurrent lung tumours after prior radical treatment poses technical challenges due to concerns of cumulative doses. SBRT of recurrent lesion could possibly provide better control benefit and reduce the toxicities because of smaller margins and tighter dose constraints. Most of the data comes from retrospective reports and a few prospective studies of both conventional and hypofractionated regimens.
Irradiation outcomes after SBRT reirradiation have yielded variable toxicity results. Reported severe ≥ grade 3 toxicity rates varied between 0% in some studies (116-122), to as high as a 10% incidence of G5 bleeding (123). Some studies noted no association between toxicities and lung dosimetric parameter, BED or overlap with prior radiotherapy fields (123-126). The risk is however increased with central tumour reirradiation.
Kilburn et al. (127) reported an incident (9.1%) of grade 5 aorta-esophageal fistula after reirradiation of a central tumour. The patient’s aorta and esophagus had received an estimated summed EQD2 of 200 and 106 Gy (128) respectively. Trovo et al. (125) reported high incidences of grade 5 (1 pneumonitis and 1 hemoptysis) and grade 4 (4 pneumonitis) toxicities after 30 Gy in 5–6 fractions in 17 patients with central tumour recurrences. The heart Dmax (mean 27 vs. 10 Gy), D5 (minimum dose to at least 5% of the heart volume) (mean 10 vs. 5 Gy) and D10 (mean 7 vs. 3 Gy) were associated with risk of severe pneumonitis. Peulen et al. (123) reported 3 deaths (10.3%) from massive bleeding and 1 grade 4 toxicity (superior vena cava stenosis and gastro-tracheal fistula) in patients with central lesion (n=11) vs. no G4–5 toxicity in peripheral lesions (n=21). Patients with a larger initial and reirradiation CTVs and a shorter interval between initial SBRT and reirradiation were at risk of more severe toxicities. Evans et al. reviewed patients who had undergone retreatment radiotherapy. Two of 35 (5.7%) patients had G5 toxicities at a median follow-up of 42 months. When 1 cm3 of aorta had a composite dose of ≥120 vs. <120 Gy, the rate of G5 aortic toxicity was 25% vs. 0% respectively (P=0.047) (129).
A team from MD Anderson have reported the largest cohort series of lung SBRT reirradiation so far. Early results after doses of 50 Gy in 4 factions, reported grade 3 toxicities of dermatitis, CW pain and brachial plexopathy (130) which were appeared related to high doses of >35 Gy to skin and ribs and >40 Gy to brachial plexus respectively. Subsequent reports (131,132) in larger cohorts reported incidences of grade 3 esophagitis, CW ulcers and cough. The reported severe pneumonitis rates was 20.8% (132). Analysis showed association between grade 3 pneumonitis and out-of-field relapse (131), ECOG 2-3 and FEV1 ≤65% before SBRT, V20 ≥30% of the composite plan, and an previous PTV spanning bilateral mediastinum (132). It is proposed that possible reason lesser rates of pneumonitis were noted in-field relapses was that previously irradiated areas are fibrotic and less susceptible to additional damage from RP. CW pain requiring narcotics was more common in patients with in-field relapses. The dose constrains used were reported (Table 3).
Table 3
Organ | Volume (cc) | Total dose/dose per fraction (Gy) |
---|---|---|
Esophagus | ≤1 | 35/8.8 |
≤10 | 30/7.5 | |
Brachial plexus | Any point | <40 |
≤1 | 35/8.8 | |
≤10 | 30/7.5 | |
Trachea | ≤1 | 35/8.8 |
≤10 | 30/7.5 | |
Main bronchus and bronchial tree | ≤1 | 40/10 |
≤10 | 35/8.8 | |
Heart | ≤1 | 40/10 |
≤10 | 35/8.8 | |
Whole lung (excluding GTV) | V20 | <20% |
V10 | <30% | |
V5 | <40% | |
Major vessels | ≤1 | 40/10 |
≤10 | 35/8.8 | |
Skin (to 5 mm) | ≤1 | 40/10 |
≤10 | 35/8.8 | |
Spinal cord | ≤1 | 20/5 |
≤10 | 15/3.8 |
NSCLC, non-small cell lung cancer; SBRT, stereotactic body radiotherapy; GTV, gross tumour volume.
Recently Chao et al. (133) reported a multi-institutional prospective trial using proton therapy for lung irradiation in 57 patients recurrent NSCLC. Median reirradiation dose prescribed was 66.6 Gy with 68% having concurrent chemotherapy. Cumulative point dose constrain to the spinal cord was 75 Gy (RBE). Forty-two percent ≥ grade 3 acute or late toxicities were reported at a median follow-up of 7.8 months. There were 6 possible or probable RT related grade 5 toxicities—bronchopulmonary hemorrhage, neutropenic sepsis, anorexia, pneumonitis, hypoxic respiratory failure/pleural effusion and tracheoesophageal fistula. Central region overlap (≥41 cm3), concurrent chemotherapy, composite mean heart dose ≥3.94 Gy and median esophagus dose of ≥12.45 Gy were associated with more toxicities.
The interpretation of results from various trials can be difficult because of heterogeneous cohorts and individually small numbers. De Bari et al. (128) and the MD Anderson (130-132) group have published their dose constraints recommendations. However, as there still many variables that cannot be accounted for e.g., disease free interval, previous radiation toxicities, individual characteristics, the OAR constraints in this setting remains poorly defined (134). Outcome results are promising but caution must still be exercised especially in reirradiation of centrally located tumors.
Conclusions
Published literature in lung SBRT have reported good local control rates with acceptable toxicity profiles. However, with the high doses per fraction used, there is an increased risk of normal tissue toxicity compared with conventionally fractionated radiation therapy. Care must be taken to minimize radiation exposure to normal structures. This is especially important for centrally located tumors. The available trial protocols and international consensus serve as good guidelines for SBRT dose constraints. Different dose-regimens have been used to increase the BED delivered and reduce toxicities. Future trials will aid in our understanding of the organ dose tolerances. Studies in biomarkers and individual patient risks are underway to better tailor our SBRT treatments.
Acknowledgments
Funding: None.
Footnote
Provenance and Peer Review: This article was commissioned by the Guest Editors (Kam-Weng Fong, Kevin Lee Min Chua) for the series “Radiotherapy in Lung Cancer” published in Journal of Xiangya Medicine. The article has undergone external peer review.
Conflicts of Interest: Both authors have completed the ICMJE uniform disclosure form (available at http://dx.doi.org/10.21037/jxym.2018.09.04). The series “Radiotherapy in Lung Cancer” was commissioned by the editorial office without any funding or sponsorship. The authors have no other conflicts of interest to declare.
Ethical Statement: The authors are accountable for all aspects of the work in ensuring that questions related to the accuracy or integrity of any part of the work are appropriately investigated and resolved.
Open Access Statement: This is an Open Access article distributed in accordance with the Creative Commons Attribution-NonCommercial-NoDerivs 4.0 International License (CC BY-NC-ND 4.0), which permits the non-commercial replication and distribution of the article with the strict proviso that no changes or edits are made and the original work is properly cited (including links to both the formal publication through the relevant DOI and the license). See: https://creativecommons.org/licenses/by-nc-nd/4.0/.
References
- Timmerman R, Papiez L, McGarry R, et al. Extracranial Stereotactic Radioablation: Results of a Phase I Study in Medically Inoperable Stage I Non-small Cell Lung Cancer. Chest 2003;124:1946-55. [Crossref] [PubMed]
- Timmerman R. Stereotactic Body Radiation Therapy for Inoperable Early Stage Lung Cancer. JAMA 2010;303:1070. [Crossref] [PubMed]
- Timmerman R, McGarry R, Yiannoutsos C, et al. Excessive toxicity when treating central tumors in a phase II study of stereotactic body radiation therapy for medically inoperable early-stage lung cancer. J Clin Oncol 2006;24:4833-9. [Crossref] [PubMed]
- Chang JY, Bezjak A, Mornex F. Stereotactic ablative radiotherapy for centrally located early stage non-small-cell lung cancer: What we have learned. J Thorac Oncol 2015;10:577-85. [Crossref] [PubMed]
- Kim MS, Kim W, Park IH, et al. Radiobiological mechanisms of stereotactic body radiation therapy and stereotactic radiation surgery. Radiat Oncol J 2015;33:265-75. [Crossref] [PubMed]
- Brown JM, Carlson DJ, Brenner DJ. The tumor radiobiology of SRS and SBRT: Are more than the 5 Rs involved? Int J Radiat Oncol Biol Phys 2014;88:254-62. [Crossref] [PubMed]
- D’Andrea M, Strolin S, Ungania S, et al. Radiobiological Optimization in Lung Stereotactic Body Radiation Therapy: Are We Ready to Apply Radiobiological Models? Front Oncol 2018;7:321. [Crossref] [PubMed]
- Liang X, Penagaricano J, Zheng D, et al. Radiobiological impact of dose calculation algorithms on biologically optimized IMRT lung stereotactic body radiation therapy plans. Radiat Oncol 2016;11:10. [Crossref] [PubMed]
- Benedict SH, Yenice KM, Followill D, et al. Stereotactic body radiation therapy: The report of AAPM Task Group 101. Med Phys 2010;37:4078-101. [Crossref] [PubMed]
- Timmerman RD, Paulus R, Pass HI, et al. RTOG 0618: Stereotactic body radiation therapy (SBRT) to treat operable early-stage lung cancer patients. J Clin Oncol 2013;31:7523.
- Videtic GM, Hu C, Singh AK, et al. A randomized phase 2 study comparing 2 stereotactic body radiation therapy schedules for medically inoperable patients with stage I peripheral non-small cell lung cancer: NRG Oncology RTOG 0915 (NCCTG N0927). Int J Radiat Oncol Biol Phys 2015;93:757-64. [Crossref] [PubMed]
- Bezjak A, Paulus R, Gaspar LE, et al. Efficacy and Toxicity Analysis of NRG Oncology/RTOG 0813 Trial of Stereotactic Body Radiation Therapy (SBRT) for Centrally Located Non-Small Cell Lung Cancer (NSCLC). Int J Radiat Oncol 2016;96:S8. [Crossref]
- Onimaru R, Shirato H, Shibata T, et al. Phase I study of stereotactic body radiation therapy for peripheral T2N0M0 non-small cell lung cancer with PTV < 100 cc using a continual reassessment method (JCOG0702). Radiother Oncol 2015;116:276-80. [Crossref] [PubMed]
- Hanna GG, Murray L, Patel R, et al. UK Consensus on Normal Tissue Dose Constraints for Stereotactic Radiotherapy. Clin Oncol (R Coll Radiol) 2018;30:5-14. [Crossref] [PubMed]
- National Cancer Institute. Common Terminology Criteria for Adverse Events (CTCAE) | Protocol Development | CTEP [Internet]. 2017 [cited 2018 Jun 21]. Available online: https://ctep.cancer.gov/protocolDevelopment/electronic_applications/ctc.htm
- Group RTO. RTOG/EORTC late radiation morbidity scoring schema. 2014-08-02]. Available online: https://www.rtog.org/ResearchAssociates/AdverseEventReporting/RTOGEORTCLateRadiationMorbidityScoringSchema.aspx
- Fakiris AJ, McGarry RC, Yiannoutsos CT, et al. Stereotactic Body Radiation Therapy for Early-Stage Non-Small-Cell Lung Carcinoma: Four-Year Results of a Prospective Phase II Study. Int J Radiat Oncol Biol Phys 2009;75:677-82. [Crossref] [PubMed]
- Henderson M, McGarry R, Yiannoutsos C, et al. Baseline Pulmonary Function as a Predictor for Survival and Decline in Pulmonary Function Over Time in Patients Undergoing Stereotactic Body Radiotherapy for the Treatment of Stage I Non-Small-Cell Lung Cancer. Int J Radiat Oncol Biol Phys 2008;72:404-9. [Crossref] [PubMed]
- Baumann P, Nyman J, Hoyer M, et al. Outcome in a prospective phase II trial of medically inoperable stage I non-small-cell lung cancer patients treated with stereotactic body radiotherapy. J Clin Oncol 2009;27:3290-6. [Crossref] [PubMed]
- Timmerman RD, Hu C, Michalski J, et al. Long-term Results of RTOG 0236: A Phase II Trial of Stereotactic Body Radiation Therapy (SBRT) in the Treatment of Patients with Medically Inoperable Stage I Non-Small Cell Lung Cancer. Int J Radiat Oncol 2014;90:S30. [Crossref]
- Stanic S, Paulus R, Timmerman RD, et al. No clinically significant changes in pulmonary function following stereotactic body radiation therapy for early- stage peripheral non-small cell lung cancer: An analysis of RTOG 0236. Int J Radiat Oncol Biol Phys 2014;88:1092-9. [Crossref] [PubMed]
- Ricardi U, Filippi AR, Guarneri A, et al. Stereotactic body radiation therapy for early stage non-small cell lung cancer: Results of a prospective trial. Lung Cancer 2010;68:72-7. [Crossref] [PubMed]
- Ricardi U, Filippi AR, Guarneri A, et al. Dosimetric predictors of radiation-induced lung injury in stereotactic body radiation therapy. Acta Oncol 2009;48:571-7. [Crossref] [PubMed]
- Le QT, Loo BW, Ho A, et al. Results of a phase I dose-escalation study using single-fraction stereotactic radiotherapy for lung tumors. J Thorac Oncol 2006;1:802-9. [Crossref] [PubMed]
- Fritz P, Kraus HJ, Mühlnickel W, et al. Stereotactic, single-dose irradiation of stage I non-small cell lung cancer and lung metastases. Radiat Oncol 2006;1:30. [Crossref] [PubMed]
- Nagata Y, Hiraoka M, Shibata T, et al. Prospective trial of stereotactic body radiation therapy for both operable and inoperable T1N0M0 non-small cell lung cancer: Japan Clinical Oncology Group Study JCOG0403. Int J Radiat Oncol Biol Phys 2015;93:989-96. [Crossref] [PubMed]
- Allibhai Z, Taremi M, Bezjak A, et al. The impact of tumor size on outcomes after stereotactic body radiation therapy for medically inoperable early-stage non-small cell lung cancer. Int J Radiat Oncol Biol Phys 2013;87:1064-70. [Crossref] [PubMed]
- McGarry RC, Papiez L, Williams M, et al. Stereotactic body radiation therapy of early-stage non-small-cell lung carcinoma: Phase I study. Int J Radiat Oncol Biol Phys 2005;63:1010-5. [Crossref] [PubMed]
- Shibamoto Y, Hashizume C, Baba F, et al. Stereotactic body radiotherapy using a radiobiology-based regimen for Stage I non-small-cell lung cancer: Five-year mature results. J Thorac Oncol 2015;10:960-4. [Crossref] [PubMed]
- Shibamoto Y, Hashizume C, Baba F, et al. Stereotactic body radiotherapy using a radiobiology-based regimen for stage i nonsmall cell lung cancer: A multicenter study. Cancer 2012;118:2078-84. [Crossref] [PubMed]
- Taremi M, Hope A, Dahele M, et al. Stereotactic body radiotherapy for medically inoperable lung cancer: Prospective, single-center study of 108 consecutive patients. Int J Radiat Oncol Biol Phys 2012;82:967-73. [Crossref] [PubMed]
- Bezjak A, Paulus R, Gaspar LE, et al. Primary Study Endpoint Analysis for NRG Oncology/RTOG 0813 Trial of Stereotactic Body Radiation Therapy (SBRT) for Centrally Located Non-Small Cell Lung Cancer (NSCLC). Int J Radiat Oncol 2016;94:5-6. [Crossref]
- Chang JY, Senan S, Paul MA, et al. Stereotactic ablative radiotherapy versus lobectomy for operable stage I non-small-cell lung cancer: A pooled analysis of two randomised trials. Lancet Oncol 2015;16:630-7. [Crossref] [PubMed]
- Lindberg K, Bergström P, Brustugun OT, et al. OA24.05 The Nordic HILUS-Trial - First Report of a Phase II Trial of SBRT of Centrally Located Lung Tumors. J Thorac Oncol 2017;12:S340. [Crossref]
- Kimura T, Nagata Y, Harada H, et al. Phase I study of stereotactic body radiation therapy for centrally located stage IA non-small cell lung cancer (JROSG10-1). Int J Clin Oncol 2017;22:849-56. [Crossref] [PubMed]
- Onimaru R, Onishi H, Shibata T, et al. Phase I study of stereotactic body radiation therapy for peripheral T2N0M0 non-small cell lung cancer (JCOG0702): Results for the group with PTV ≥100 cc. Radiother Oncol 2017;122:281-5. [Crossref] [PubMed]
- Nagata Y, Takayama K, Matsuo Y, et al. Clinical outcomes of a phase I/II study of 48 Gy of stereotactic body radiotherapy in 4 fractions for primary lung cancer using a stereotactic body frame. Int J Radiat Oncol Biol Phys 2005;63:1427-31. [Crossref] [PubMed]
- Bral S, Gevaert T, Linthout N, et al. Prospective, risk-adapted strategy of stereotactic body radiotherapy for early-stage non-small-cell lung cancer: Results of a phase II trial. Int J Radiat Oncol Biol Phys 2011;80:1343-9. [Crossref] [PubMed]
- Grills IS, Hope AJ, Guckenberger M, et al. A collaborative analysis of stereotactic lung radiotherapy outcomes for early-stage non-small-cell lung cancer using daily online cone-beam computed tomography image-guided radiotherapy. J Thorac Oncol 2012;7:1382-93. [Crossref] [PubMed]
- Yamashita H, Nakagawa K, Nakamura N, et al. Exceptionally high incidence of symptomatic grade 2-5 radiation pneumonitis after stereotactic radiation therapy for lung tumors. Radiat Oncol 2007;2:21. [Crossref] [PubMed]
- Lagerwaard FJ, Haasbeek CJ, Smit EF, et al. Outcomes of Risk-Adapted Fractionated Stereotactic Radiotherapy for Stage I Non-Small-Cell Lung Cancer. Int J Radiat Oncol Biol Phys 2008;70:685-92. [Crossref] [PubMed]
- Kyas I, Hof H, Debus J, et al. Prediction of radiation-induced changes in the lung after stereotactic body radiation therapy of non-small-cell lung cancer. Int J Radiat Oncol Biol Phys 2007;67:768-74. [Crossref] [PubMed]
- Hope AJ, Lindsay PE, El Naqa I, et al. Modeling radiation pneumonitis risk with clinical, dosimetric, and spatial parameters. Int J Radiat Oncol Biol Phys 2006;65:112-24. [Crossref] [PubMed]
- Yorke ED, Jackson A, Rosenzweig KE, et al. Dose-volume factors contributing to the incidence of radiation pneumonitis in non-small-cell lung cancer patients treated with three-dimensional conformal radiation therapy. Int J Radiat Oncol Biol Phys 2002;54:329-39. [Crossref] [PubMed]
- West J. Respiratory physiology: the essentials. 2005.
- Barriger RB, Forquer JA, Brabham JG, et al. A dose-volume analysis of radiation pneumonitis in non-small cell lung cancer patients treated with stereotactic body radiation therapy. Int J Radiat Oncol Biol Phys 2012;82:457-62. [Crossref] [PubMed]
- Inoue T, Katoh N, Onimaru R, et al. Stereotactic body radiotherapy using gated radiotherapy with real-time tumor-tracking for stage I non-small cell lung cancer. Radiat Oncol 2013;8:69. [Crossref] [PubMed]
- Matsuo Y, Shibuya K, Nakamura M, et al. Dose-volume metrics associated with radiation pneumonitis after stereotactic body radiation therapy for lung cancer. Int J Radiat Oncol Biol Phys 2012;83:e545-9. [Crossref] [PubMed]
- Ueki N, Matsuo Y, Togashi Y, et al. Impact of pretreatment interstitial lung disease on radiation pneumonitis and survival after stereotactic body radiation therapy for lung cancer. J Thorac Oncol 2015;10:116-25. [Crossref] [PubMed]
- Chang JY, Liu H, Balter P, et al. Clinical outcome and predictors of survival and pneumonitis after stereotactic ablative radiotherapy for stage I non-small cell lung cancer. Radiat Oncol 2012;7:152. [Crossref] [PubMed]
- Ong CL, Palma D, Verbakel WF, et al. Treatment of large stage I-II lung tumors using stereotactic body radiotherapy (SBRT): Planning considerations and early toxicity. Radiother Oncol 2010;97:431-6. [Crossref] [PubMed]
- Bongers EM, Botticella A, Palma DA, et al. Predictive parameters of symptomatic radiation pneumonitis following stereotactic or hypofractionated radiotherapy delivered using volumetric modulated arcs. Radiother Oncol 2013;109:95-9. [Crossref] [PubMed]
- Appelt AL, Vogelius IR, Farr KP, et al. Towards individualized dose constraints: Adjusting the QUANTEC radiation pneumonitis model for clinical risk factors. Acta Oncol 2014;53:605-12. [Crossref] [PubMed]
- Vogelius IR, Bentzen SM. A literature-based meta-analysis of clinical risk factors for development of radiation induced pneumonitis. Acta Oncol 2012;51:975-83. [Crossref] [PubMed]
- Sause WT, Scott C, Taylor S, et al. Radiation Therapy Oncology Group (RTOG) 88-08 and Eastern Cooperative Oncology Group (ECOG) 4588: Preliminary Results of a Phase III Trial in Regionally Advanced, Unresectable Non-Small-Cell Lung Cancer. J Natl Cancer Inst 1995;87:198-205. [Crossref] [PubMed]
- Takeda A, Ohashi T, Kunieda E, et al. Comparison of clinical, tumour-relatedand dosimetric factors in grade 0-1, grade2 and grade 3 radiation pneumonitis after stereotactic body radiotherapy for lung tumours. Br J Radiol 2012;85:636-42. [Crossref] [PubMed]
- Takeda A, Kunieda E, Ohashi T, et al. Severe COPD is correlated with mild radiation pneumonitis following stereotactic body radiotherapy. Chest 2012;141:858-66. [Crossref] [PubMed]
- Bjermer L, Cai Y, Nilsson K, et al. Tobacco smoke exposure suppresses radiation-induced inflammation in the lung: A study of bronchoalveolar lavage and ultrastructural morphology in the rat. Eur Respir J 1993;6:1173-80. [PubMed]
- Bhattathiri VN. Possible role of plasma GSH in modulating smoking related radiation pneumonitis. Radiother Oncol 1999;51:291-2. [PubMed]
- Jin H, Tucker SL, Liu HH, et al. Dose-volume thresholds and smoking status for the risk of treatment-related pneumonitis in inoperable non-small cell lung cancer treated with definitive radiotherapy. Radiother Oncol 2009;91:427-32. [Crossref] [PubMed]
- Pang Q, Wei Q, Xu T, et al. Functional promoter variant rs2868371 of HSPB1 is associated with risk of radiation pneumonitis after chemoradiation for non-small cell lung cancer. Int J Radiat Oncol Biol Phys 2013;85:1332-9. [Crossref] [PubMed]
- Mak RH, Alexander BM, Asomaning K, et al. A single-nucleotide polymorphism in the methylene tetrahydrofolate reductase (MTHFR) gene is associated with risk of radiation pneumonitis in lung cancer patients treated with thoracic radiation therapy. Cancer 2012;118:3654-65. [Crossref] [PubMed]
- Yin M, Liao Z, Yuan X, et al. Polymorphisms of the vascular endothelial growth factor gene and severe radiation pneumonitis in non-small cell lung cancer patients treated with definitive radiotherapy. Cancer Sci 2012;103:945-50. [Crossref] [PubMed]
- Xiong H, Liao Z, Liu Z, et al. ATM polymorphisms predict severe radiation pneumonitis in patients with non-small cell lung cancer treated with definitive radiation therapy. Int J Radiat Oncol Biol Phys 2013;85:1066-73. [Crossref] [PubMed]
- Zhang L, Yang M, Bi N, et al. ATM polymorphisms are associated with risk of radiation-induced pneumonitis. Int J Radiat Oncol Biol Phys 2010;77:1360-8. [Crossref] [PubMed]
- Zhang L, Wang L, Yang M, et al. Association of DNA Repair and Inflammatory Cytokines Gene Polymorphisms With Radiation-Induced Toxicity in Lung Cancer. Int J Radiat Oncol 2007;69:S60-1. [Crossref]
- Guckenberger M, Kestin LL, Hope AJ, et al. Is there a lower limit of pretreatment pulmonary function for safe and effective stereotactic body radiotherapy for early-stage non-small cell lung cancer? J Thorac Oncol 2012;7:542-51. [Crossref] [PubMed]
- Stephans KL, Djemil T, Reddy CA, et al. Comprehensive analysis of pulmonary function test (PFT) changes after stereotactic body radiotherapy (SBRT) for stage I lung cancer in medically inoperable patients. J Thorac Oncol 2009;4:838-44. [Crossref] [PubMed]
- Chen H, Senan S, Nossent EJ, et al. Treatment-Related Toxicity in Patients With Early-Stage Non-Small Cell Lung Cancer and Coexisting Interstitial Lung Disease: A Systematic Review. Int J Radiat Oncol Biol Phys 2017;98:622-31. [Crossref] [PubMed]
- Bahig H, Filion E, Vu T, et al. Severe radiation pneumonitis after lung stereotactic ablative radiation therapy in patients with interstitial lung disease. Pract Radiat Oncol 2016;6:367-74. [Crossref] [PubMed]
- Sanuki N, Ono A, Komatsu E, et al. Association of Computed Tomography-detected Pulmonary Interstitial Changes with Severe Radiation Pneumonitis for Patients Treated with Thoracic Radiotherapy. J Radiat Res 2012;53:110-6. [Crossref] [PubMed]
- Lee CE, Prabhu V, Slevin NJ. Collagen Vascular Diseases and Enhanced Radiotherapy-induced Normal Tissue Effects - a Case Report and a Review of Published Studies. Clin Oncol (R Coll Radiol) 2011;23:73-8. [Crossref] [PubMed]
- Chaudhuri AA, Binkley MS, Rigdon J, et al. Pre-treatment non-target lung FDG-PET uptake predicts symptomatic radiation pneumonitis following Stereotactic Ablative Radiotherapy (SABR)Pre-treatment non-target lung FDG-PET uptake predicts radiation pneumonitis after SABR. Radiother Oncol 2016;119:454-60. [Crossref] [PubMed]
- Yuan X, Liao Z, Liu Z, et al. Single Nucleotide Polymorphism at rs1982073:T869C of the TGF β 1 Gene Is Associated With the Risk of Radiation Pneumonitis in Patients With Non-Small-Cell Lung Cancer Treated With Definitive Radiotherapy. J Clin Oncol 2009;27:3370-8. [Crossref] [PubMed]
- Anscher MS, Kong FM, Andrews K, et al. Plasma transforming growth factor β1as a predictor of radiation pneumonitis. Int J Radiat Oncol Biol Phys 1998;41:1029-35. [Crossref] [PubMed]
- Zhao L, Sheldon K, Chen M, et al. The predictive role of plasma TGF-β1 during radiation therapy for radiation-induced lung toxicity deserves further study in patients with non-small cell lung cancer. Lung Cancer 2008;59:232-9. [Crossref] [PubMed]
- Chen Y, Williams J, Ding I, et al. Radiation pneumonitis and early circulatory cytokine markers. Semin Radiat Oncol 2002;12:26-33. [Crossref] [PubMed]
- Chen Y, Rubin P, Williams J, et al. Circulating IL-6 as a predictor of radiation pneumonitis. Int J Radiat Oncol Biol Phys 2001;49:641-8. [Crossref] [PubMed]
- Stenmark MH, Cai XW, Shedden K, et al. Combining physical and biologic parameters to predict radiation-induced lung toxicity in patients with non-small-cell lung cancer treated with definitive radiation therapy. Int J Radiat Oncol Biol Phys 2012;84:e217-22. [Crossref] [PubMed]
- Yamashita H, Kobayashi-Shibata S, Terahara A, et al. Prescreening based on the presence of CT-scan abnormalities and biomarkers (KL-6 and SP-D) may reduce severe radiation pneumonitis after stereotactic radiotherapy. Radiat Oncol 2010;5:32. [Crossref] [PubMed]
- Iwata H, Shibamoto Y, Baba F, et al. Correlation between the serum KL-6 level and the grade of radiation pneumonitis after stereotactic body radiotherapy for stage i lung cancer or small lung metastasis. Radiother Oncol 2011;101:267-70. [Crossref] [PubMed]
- Darby SC, Cutter DJ, Boerma M, et al. Radiation-Related Heart Disease: Current Knowledge and Future Prospects. Int J Radiat Oncol Biol Phys 2010;76:656-65. [Crossref] [PubMed]
- Darby SC, Ewertz M, McGale P, et al. Risk of Ischemic Heart Disease in Women after Radiotherapy for Breast Cancer. N Engl J Med 2013;368:987-98. [Crossref] [PubMed]
- Bradley JD, Paulus R, Komaki R, et al. Standard-dose versus high-dose conformal radiotherapy with concurrent and consolidation carboplatin plus paclitaxel with or without cetuximab for patients with stage IIIA or IIIB non-small-cell lung cancer (RTOG 0617): A randomised, two-by-two factorial phase 3 study. Lancet Oncol 2015;16:187-99. [Crossref] [PubMed]
- Bradley JD, Hu C, Komaki RU, et al. Long-Term Results of RTOG 0617: A Randomized Phase 3 Comparison of Standard Dose Versus High Dose Conformal Chemoradiation Therapy +/- Cetuximab for Stage III NSCLC. Int J Radiat Oncol 2017;99:S105. [Crossref]
- Stam B, Peulen H, Guckenberger M, et al. Dose to heart substructures is associated with non-cancer death after SBRT in stage I-II NSCLC patients. Radiother Oncol 2017;123:370-5. [Crossref] [PubMed]
- Evans JD, Gomez DR, Chang JY, et al. Cardiac18F-fluorodeoxyglucose uptake on positron emission tomography after thoracic stereotactic body radiation therapy. Radiother Oncol 2013;109:82-8. [Crossref] [PubMed]
- Bonomo P, Livi L, Rampini A, et al. Radioterapia stereotassica extracranica per metastasi cardiache e paracardiache: esperienza dell’Università di Firenze. Radiol Medica 2013;118:1055-65. [Crossref]
- Xue J, Kubicek G, Patel A, et al. Validity of Current Stereotactic Body Radiation Therapy Dose Constraints for Aorta and Major Vessels. Seminars in Radiation Oncology 2016;26:135-9. [Crossref] [PubMed]
- Chi A, Nguyen NP, Komaki R. The Potential Role of Respiratory Motion Management and Image Guidance in the Reduction of Severe Toxicities Following Stereotactic Ablative Radiation Therapy for Patients with Centrally Located Early Stage Non-Small Cell Lung Cancer or Lung Metastases. Front Oncol 2014;4:151. [Crossref] [PubMed]
- Kelsey CR, Kahn D, Hollis DR, et al. Radiation-induced narrowing of the tracheobronchial tree: An in-depth analysis. Lung Cancer 2006;52:111-6. [Crossref] [PubMed]
- Song SY, Choi W, Shin SS, et al. Fractionated stereotactic body radiation therapy for medically inoperable stage I lung cancer adjacent to central large bronchus. Lung Cancer 2009;66:89-93. [Crossref] [PubMed]
- Karlsson K, Nyman J, Baumann P, et al. Retrospective cohort study of bronchial doses and radiation-induced atelectasis after stereotactic body radiation therapy of lung tumors located close to the bronchial tree. Int J Radiat Oncol Biol Phys 2013;87:590-5. [Crossref] [PubMed]
- Duijm M, Schillemans W, Aerts JG, et al. Dose and Volume of the Irradiated Main Bronchi and Related Side Effects in the Treatment of Central Lung Tumors With Stereotactic Radiotherapy. Semin Radiat Oncol 2016;26:140-8. [Crossref] [PubMed]
- Rowe BP, Boffa DJ, Wilson LD, et al. Stereotactic body radiotherapy for central lung tumors. J Thorac Oncol 2012;7:1394-9. [Crossref] [PubMed]
- Milano MT, Chen Y, Katz AW, et al. Central thoracic lesions treated with hypofractionated stereotactic body radiotherapy. Radiother Oncol 2009;91:301-6. [Crossref] [PubMed]
- Oshiro Y, Aruga T, Tsuboi K, et al. Stereotactic body radiotherapy for lung tumors at the pulmonary hilum. Strahlenther Onkol 2010;186:274-9. [Crossref] [PubMed]
- Corradetti MN, Haas AR, Rengan R. Central-Airway Necrosis after Stereotactic Body-Radiation Therapy. N Engl J Med 2012;366:2327-9. [Crossref] [PubMed]
- Onimaru R, Shirato H, Shimizu S, et al. Tolerance of organs at risk in small-volume, hypofractionated, image-guided radiotherapy for primary and metastatic lung cancers. Int J Radiat Oncol Biol Phys 2003;56:126-35. [Crossref] [PubMed]
- Cox BW, Jackson A, Hunt M, et al. Esophageal toxicity from high-dose, single-fraction paraspinal stereotactic radiosurgery. Int J Radiat Oncol Biol Phys 2012;83:e661-7. [Crossref] [PubMed]
- Abelson JA, Murphy JD, Loo BW, et al. Esophageal tolerance to high-dose stereotactic ablative radiotherapy. Dis Esophagus 2012;25:623-9. [Crossref] [PubMed]
- Senthi S, Haasbeek CJ, Slotman BJ, et al. Outcomes of stereotactic ablative radiotherapy for central lung tumours: A systematic review. Radiother Oncol 2013;106:276-82. [Crossref] [PubMed]
- Adebahr S, Collette S, Shash E, et al. LungTech, an EORTC Phase II trial of stereotactic body radiotherapy for centrally located lung tumours: A clinical perspective. Br J Radiol 2015;88:20150036. [Crossref] [PubMed]
- Mutter RW, Liu F, Abreu A, et al. Dose-volume parameters predict for the development of chest wall pain after stereotactic body radiation for lung cancer. Int J Radiat Oncol Biol Phys 2012;82:1783-90. [Crossref] [PubMed]
- Andolino DL, Forquer JA, Henderson MA, et al. Chest wall toxicity after stereotactic body radiotherapy for malignant lesions of the lung and liver. Int J Radiat Oncol Biol Phys 2011;80:692-7. [Crossref] [PubMed]
- Creach KM, El Naqa I, Bradley JD, et al. Dosimetric predictors of chest wall pain after lung stereotactic body radiotherapy. Radiother Oncol 2012;104:23-7. [Crossref] [PubMed]
- Stephans KL, Djemil T, Tendulkar RD, et al. Prediction of chest wall toxicity from lung stereotactic body radiotherapy (SBRT). Int J Radiat Oncol Biol Phys 2012;82:974-80. [Crossref] [PubMed]
- Ricco A, Davis J, Rate W, et al. Lung metastases treated with stereotactic body radiotherapy: The RSSearchï¿1/2 patient Registry’s experience. Radiat Oncol 2017;12:35. [Crossref] [PubMed]
- Welsh J, Thomas J, Shah D, et al. Obesity increases the risk of chest wall pain from thoracic stereotactic body radiation therapy. Int J Radiat Oncol Biol Phys 2011;81:91-6. [Crossref] [PubMed]
- Hoppe BS, Laser B, Kowalski AV, et al. Acute Skin Toxicity Following Stereotactic Body Radiation Therapy for Stage I Non-Small-Cell Lung Cancer: Who’s at Risk? Int J Radiat Oncol Biol Phys 2008;72:1283-6. [Crossref] [PubMed]
- Bongers EM, Haasbeek CJ, Lagerwaard FJ, et al. Incidence and risk factors for chest wall toxicity after risk-adapted stereotactic radiotherapy for early-stage lung cancer. J Thorac Oncol 2011;6:2052-7. [Crossref] [PubMed]
- Li Q, Swanick CW, Allen PK, et al. Stereotactic ablative radiotherapy (SABR) using 70 Gy in 10 fractions for non-small cell lung cancer: Exploration of clinical indications. Radiother Oncol 2014;112:256-61. [Crossref] [PubMed]
- Schierle C, Winograd JM. Radiation-Induced Brachial Plexopathy: Review. Complication Without a Cure. J Reconstr Microsurg 2004;20:149-52. [Crossref] [PubMed]
- Chang JY, Li QQ, Xu QY, et al. Stereotactic ablative radiation therapy for centrally located early stage or isolated parenchymal recurrences of non-small cell lung cancer: How to fly in a “no fly zone.” Int J Radiat Oncol Biol Phys 2014;88:1120-8. [Crossref] [PubMed]
- Forquer JA, Fakiris AJ, Timmerman RD, et al. Brachial plexopathy from stereotactic body radiotherapy in early-stage NSCLC: Dose-limiting toxicity in apical tumor sites. Radiother Oncol 2009;93:408-13. [Crossref] [PubMed]
- Coon D, Gokhale AS, Burton SA, et al. Fractionated stereotactic body radiation therapy in the treatment of primary, recurrent, and metastatic lung tumors: The role of positron emission tomography/computed tomography - based treatment planning. Clin Lung Cancer 2008;9:217-21. [Crossref] [PubMed]
- Ceylan C, Hamacl A, Ayata H, et al. Re-Irradiation of Locoregional NSCLC Recurrence Using Robotic Stereotactic Body Radiotherapy. Oncol Res Treat 2017;40:207-14. [Crossref] [PubMed]
- Seung SK, Solhjem M. Salvage SBRT for Previously Irradiated Lung Cancer. J Cancer Ther 2011;02:190-5. [Crossref]
- Trakul N, Harris JP, Le QT, et al. Stereotactic Ablative Radiotherapy for Reirradiation of Locally Recurrent Lung Tumors. J Thorac Oncol 2012;7:1462-5. [Crossref] [PubMed]
- Meijneke TR, Petit SF, Wentzler D, et al. Reirradiation and stereotactic radiotherapy for tumors in the lung: Dose summation and toxicity. Radiother Oncol 2013;107:423-7. [Crossref] [PubMed]
- Hearn JW, Videtic GM, Djemil T, et al. Salvage stereotactic body radiation therapy (SBRT) for local failure after primary lung SBRT. Int J Radiat Oncol Biol Phys 2014;90:402-6. [Crossref] [PubMed]
- Patel NR, Lanciano R, Sura K, et al. Stereotactic body radiotherapy for re-irradiation of lung cancer recurrence with lower biological effective doses. J Radiat Oncol 2015;4:65-70. [Crossref] [PubMed]
- Peulen H, Karlsson K, Lindberg K, et al. Toxicity after reirradiation of pulmonary tumours with stereotactic body radiotherapy. Radiother Oncol 2011;101:260-6. [Crossref] [PubMed]
- Caivano D, Valeriani M, De Matteis S, et al. Re-irradiation in lung disease by SBRT: A retrospective, single institutional study. Radiat Oncol 2018;13:87. [Crossref] [PubMed]
- Trovo M, Minatel E, Durofil E, et al. Stereotactic body radiation therapy for re-irradiation of persistent or recurrent non-small cell lung cancer. Int J Radiat Oncol Biol Phys 2014;88:1114-9. [Crossref] [PubMed]
- Reyngold M, Wu AJ, McLane A, et al. Toxicity and outcomes of thoracic re-irradiation using stereotactic body radiation therapy (SBRT). Radiat Oncol 2013;8:99. [Crossref] [PubMed]
- Kilburn JM, Kuremsky JG, Blackstock AW, et al. Thoracic re-irradiation using stereotactic body radiotherapy (SBRT) techniques as first or second course of treatment. Radiother Oncol 2014;110:505-10. [Crossref] [PubMed]
- De Bari B, Filippi AR, Mazzola R, et al. Available evidence on re-irradiation with stereotactic ablative radiotherapy following high-dose previous thoracic radiotherapy for lung malignancies. Cancer Treat Rev 2015;41:511-8. [Crossref] [PubMed]
- Evans JD, Gomez DR, Amini A, et al. Aortic dose constraints when reirradiating thoracic tumors. Radiother Oncol 2013;106:327-32. [Crossref] [PubMed]
- Chang JY, Balter PA, Dong L, et al. Stereotactic Body Radiation Therapy in Centrally and Superiorly Located Stage I or Isolated Recurrent Non-Small-Cell Lung Cancer. Int J Radiat Oncol Biol Phys 2008;72:967-71. [Crossref] [PubMed]
- Kelly P, Balter PA, Rebueno N, et al. Stereotactic body radiation therapy for patients with lung cancer previously treated with thoracic radiation. Int J Radiat Oncol Biol Phys 2010;78:1387-93. [Crossref] [PubMed]
- Liu H, Zhang X, Vinogradskiy YY, et al. Predicting radiation pneumonitis after stereotactic ablative radiation therapy in patients previously treated with conventional thoracic radiation therapy. Int J Radiat Oncol Biol Phys 2012;84:1017-23. [Crossref] [PubMed]
- Chao HH, Berman AT, Simone CB, et al. Multi-Institutional Prospective Study of Reirradiation with Proton Beam Radiotherapy for Locoregionally Recurrent Non-Small Cell Lung Cancer. J Thorac Oncol 2017;12:281-92. [Crossref] [PubMed]
- Rulach R, Hanna GG, Franks K, et al. Re-irradiation for Locally Recurrent Lung Cancer: Evidence, Risks and Benefits. Clin Oncol 2018;30:101-9. [Crossref] [PubMed]
Cite this article as: Chia BSH, Master Z. Pitfalls in lung stereotactic body radiotherapy—a review of organ toxicities and dose constraints. J Xiangya Med 2018;3:36.