Current perspectives of recurrence and progression in hepatocellular carcinoma
Introduction
Hepatocellular carcinoma (HCC) accounts for the second place of cancer deaths, and is responsible for about 746,000 deaths per year worldwide (1). Five-year recurrence rates after liver transplantation in HCC patients meeting Milan or University of California San Francisco criteria were 5.8% and 14.3%, respectively (2). As shown in Table 1, several recent studies showed significant relationship between high Edmondson-Steiner grades (III–IV) and occurrence of recurrence, which increased the risk of development of tumor recurrence in patients with HCC (3-7). Despite extensive clinical as well as basic research efforts, the invasion and metastasis of HCC are the main reason of operative failure and the main factor affecting survival rate. Therefore, understanding mechanisms of HCC are even more important. In this review, we assessed these mechanisms that promote the recurrence and progression of HCC.
Table 1
Reference | Edmondson-Steiner grade | Univariate analysis | Multivariate analysis | |||
---|---|---|---|---|---|---|
Hazard ratio (95% CI) | P | Hazard ratio (95% CI) | P | |||
Xiao et al. (3) | Low grade (I–II) vs. high grade (III–IV) | 1.75 (0.88–2.36) | 0.021 | 1.66 (0.71–3.15) | Non-significant | |
Lee K et al. (4) | Low grade (I–II) vs. high grade (III–IV) | – | – | 1.265 (1.017–1.573) | 0.034 | |
Zhou et al. (5) | Low grade (I–II) vs. high grade (III–IV) | 5.440 (3.337–8.867) | <0.001 | |||
Perea Del Pozo et al. (6) | Low grade (I–II) vs. high grade (III–IV) | Not determined (0.759–8.777) | 0.129 | |||
Zhu et al. (7) | Low grade (I–II) vs. high grade (III–IV) | 1.866 (1.125–3.096) | 0.016 |
Liver cancer stem cells
As previously reported, liver cancer stem cells have been identified by several biomarkers such as EpCAM (8), CD24 (9), CD90 (10), and CD133 (11), and these liver cancer stem cells are relatively resistant to chemotherapy and capable of self-renewal. CD24 overexpression is associated with increased risk for tumor recurrence in HCC, and shorter disease-free survival. It is thought that CD24+ HCC cells functioned to initiate tumor self-renewal and growth by Stat3-mediated Nanog up-regulation (9). CD133+ liver cancer stem cell was promoted to self-renewal and growth by miR-130b, in part, through silencing p53-inducible nuclear protein (11).
Biological activity of liver cancer cells
Almost 32% of all human liver tumor cases show somatic mutation of p53, suggesting that tumor suppressor p53 has important roles in hepatocarcinogenesis (12). MDM2 overexpression is associated with increased risk for damaged cells to escape the cell-cycle checkpoint and become carcinogenic through Inhibiting p53 (13). Furthermore, the combination of p53 and MDM2 polymorphisms correlates with tumor progression and poor prognosis in postoperative patients with clinically (14). Human miR-146a is embedded on chromosome 5q34, which is a region that is often deleted in liver cancer (15), and miR-146a expression was negative correlated with increased HAb18G, vascular endothelial growth factor (VEGF), NF-κB p65 and beneficial prognosis of HCC (16).
Both β-catenin mutations and activation of H-ras signaling pathways, such as overexpression of insulin-like growth factor II or transforming growth factor α, occurs in human HCC (17) and β-catenin accumulation has been correlated with HCC progression (18). Moreover, Wnt-transforming growth factor-β-class plays a critical role in transforming growth factor-β activation, vascular invasion, and postoperative recurrence in patients with HCC (19). We previously investigated the expression of multiple genes in hepatitis B virus-related liver cancer and adjacent normal tissues by use of a cDNA microarray assay to establish the gene profile, and RT-PCR to measure expression of candidate genes. We found that normal liver tissues had low expression of Transmembrane 4 superfamily member 1 (TM4SF1), but liver cancer had high expression of TM4SF1, and suggest that TM4SF1 overexpression correlates with tumor progression and poor prognosis in patients with HCC (20,21).
Cyclin D1 can form complexes with cyclin-dependent kinase 4 and cyclin-dependent kinase 6. These complexes have protein kinase activity and regulate the transcription of genes related to DNA replication in a positive feedback loop. This promotes cell progression from the G1 phase to S phase and leads cell proliferation (22). In HCC, numerous studies have reported cyclin D1 overexpression, and a relationship of this overexpression with cell differentiation, invasive growth, and metastasis (23,24). Cyclin D1 is overexpressed in liver cancer, and promotes the metastasis of liver cancer (25). Thus, the upregulation of Cyclin D1 might play an important role in cancer cell invasion (26,27).
Proliferating cell nuclear antigen (PCNA) is necessary for DNA synthesis in eukaryotic cells. PCNA is an accessory protein of DNA polymerase, which is indispensable for DNA synthesis whose expression is correlative with cell proliferation and progression of the cell cycle. Thus, previous studies have used PCNA as a marker of cell proliferation (28). PCNA is not expressed in the G0 phase, first appears late in the G1 phase, reaches a peak in the S phase, and declines in the M phase. Malignancies are characterized by aberrant cell proliferation and thus PCNA may be used as an indicator of cell proliferation and biological activity of liver cancer cells (29). In liver cancer patients, PCNA overexpression is correlated with poor prognosis in postoperative patients (30). Moreover, PCNA expression is significantly higher in involved than in uninvolved vessels, and may be related to poor cell differentiation, high malignancy, and susceptibility to vascular involvement (31,32).
Studies of nude mice showed that a heterozygous mutation of the Beclin-1 gene increased cell proliferation and reduced autophagy, and that these Beclin-1+/− mice had a high incidence of liver cancer (33). In addition, after introduction of Beclin-1 into nude mice, liver cancer cells exhibited increased autophagy, loss of the malignant phenotype, reduced cell proliferation, decreased colony formation rate, and reduced susceptibility to tumorigenesis (34).
Autophagy also plays important role in tumorigenesis. In particular, cancer cells with deficient apoptosis may counteract the incipient necrosis and inflammation via autophagy, and deficient autophagy is positively associated with malignant proliferation of cancers (35). Microtubule-associated proteins 1A/1B light chain 3A (LC3) is widely expressed in different types of cells, and may be a specific marker of autophagy (36). After surgery of liver cancer patients, elevated LC3 expression is associated with longer survival (37).
On the other side, autophagy also promotes the survival of cancer cells (38). Thus, autophagy is a double-edged sword: it inhibits tumorigenesis in the early phase of tumorigenesis, but it also allows cancer cells to adapt to their adverse metabolic environment and thereby improves their survival. In other words, autophagy has different roles in the different phases of tumorigenesis and in different cancers.
miR-122 overexpression in HCC cells stimulates the expression of E-cadherin, occluding, and α-catenin, and inhibits the expression of mesenchymal proteins (fibronectin and vimentin), RhoA/Rock pathway inactivation, and invasion of HCC cells (39). Loss of miR-1258 contributes to carcinogenesis and progression of HCC through targeting CDC28 protein kinase regulatory subunit 1B and associated with poor patients’ survival (40). Recent studies showed that MTHFR C677T polymorphism was significantly associated with susceptibility to HCC in Chinese population, and MTHFR A1298C polymorphism was conversely associated with liver cancer risk in Chinese population (41). Decreased expression of fructose-1,6-bisphosphatase in HCC contributed to tumor progression and poor prognosis by altering glucose metabolism (42). HBx expression in liver cancer region of HCC is accompanied by the specific synthesis of sialyl lewis A and induced expression of N-acetylglucosamine-β1-3 galactosyltransferase V gene associated with the initial synthesis of sialyl lewis A, and N-acetylglucosamine-β1-3 galactosyltransferase V silencing suppressed hematogenous cancer cell adhesion to endothelial cells for cancer metastasis (43). Intercellular adhesion molecule-1 has the capability to induce the adhesion between cancer cells, and overexpression of intercellular adhesion molecule-1 correlates with HCC clinical tumor-node-metastasis stage, portal vein tumor thrombus, distant metastasis, and recurrence (44).
Vascular endothelial growth factor and its receptor in HCC
Proteins in the VEGF family, which are synthesized and secreted by normal cells and cancer cells, are important pro-angiogenic factors (45). VEGF binds to their receptors and promote the proliferation and metastasis of cancer cells by several mechanisms: mediation of the adhesion of cancer cells to endothelial cells and migration of cancer cells across endothelial cells; promotion of angiogenesis; phosphorylation and inactivation of pro-apoptotic proteins, leading to inhibition of apoptosis in cancer cells; and promotion of the differentiation and proliferation of cancer cells (46-49). VEGF significantly increases the activities of matrix metalloproteinase 2 (MMP-2) and MMP-9 in liver cancer cells, and inhibition of VEGF almost completely blocks activation of these MMPs in liver cancer cells (50). In addition, VEGF may induce expression of MMP-9 and act synergistically with MMP-9 to induce angiogenesis in cancer, leading to cancer progression and metastasis. These findings suggest that proteins in the VEGF family play key roles in the recurrence and metastasis of liver cancer (51).
Urokinase-type plasminogen activator (uPA) and its regulatory factors
The extracellular matrix (ECM) also has a role in the angiogenesis and metastasis of cancers. In particular, degradation of the vascular basement membrane and the ECM is required for cancer angiogenesis (52). uPA and its inhibitor (PAI), and MMPs and their inhibitors (tissue inhibitors of metalloproteinases, TIMPs) play important roles in degradation of the ECM and basement membrane (53). uPA is a serine protease that binds to specific receptors on the cell membrane and activates plasminogen to plasmin; it also promotes the degradation of ECM (including laminin, fibronectin, proteoglycans, and type IV collagen) and the vascular basement membrane, leading to invasion and metastasis of cancer cells. uPA and plasminogen activator inhibitor-1 (PAI-1) have high expression in liver cancers. In particular, liver cancer patients with portal vein tumor thrombi and metastasis have significantly higher expression of uPA and PAI-1 in tumor cells than in adjacent normal tissues and controls. Liver cancers that are positive for uPA, uPA receptor, and PAI-1 are more susceptible to invasion and metastasis (Figure 1) (54).
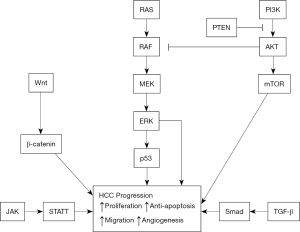
Conclusions
In summary, the invasion and metastasis of HCC is a complex process involving multiple steps and factors. For new therapeutic strategies, a better understanding of the molecular mechanisms of development and progression of HCC is required. Characterizing the molecular mechanisms underlying HCC invasion and metastasis would help to improve the strategies for liver cancer therapy.
Acknowledgments
Funding: This work was supported by the Natural Science Foundation of Hunan Province of China (13JJ6009 to YK Huang) and the key development program of Hunan Province of China (2015JC3003 to F Qiu).
Footnote
Conflicts of Interest: Both authors have completed the ICMJE uniform disclosure form (available at http://dx.doi.org/10.21037/jxym.2017.09.01). The authors have no conflicts of interest to declare.
Ethical Statement: The authors are accountable for all aspects of the work in ensuring that questions related to the accuracy or integrity of any part of the work are appropriately investigated and resolved.
Open Access Statement: This is an Open Access article distributed in accordance with the Creative Commons Attribution-NonCommercial-NoDerivs 4.0 International License (CC BY-NC-ND 4.0), which permits the non-commercial replication and distribution of the article with the strict proviso that no changes or edits are made and the original work is properly cited (including links to both the formal publication through the relevant DOI and the license). See: https://creativecommons.org/licenses/by-nc-nd/4.0/.
References
- Torre LA, Bray F, Siegel RL, et al. Global cancer statistics, 2012. CA Cancer J Clin 2015;65:87-108. [Crossref] [PubMed]
- Unek T, Karademir S, Arslan NC, et al. Comparison of Milan and UCSF criteria for liver transplantation to treat hepatocellular carcinoma. World J Gastroenterol 2011;17:4206-12. [Crossref] [PubMed]
- Xiao S, Liu L, Fang M, et al. BPTF Associated with EMT Indicates Negative Prognosis in Patients with Hepatocellular Carcinoma. Dig Dis Sci 2015;60:910-8. [Crossref] [PubMed]
- Lee K, Lee KB, Yi NJ, et al. Prognosis of Hepatocellular Carcinoma after Liver Transplantation: Comparative Analysis with Partial Hepatectomy. J Pathol Transl Med 2017;51:79-86. [Crossref] [PubMed]
- Zhou L, Rui JA, Wang SB, et al. Clinicopathological predictors of poor survival and recurrence after curative resection in hepatocellular carcinoma without portal vein tumor thrombosis. Pathol Oncol Res 2015;21:131-8. [Crossref] [PubMed]
- Perea Del Pozo E, Bernal Bellido C, Sendin Matin M, et al. Recurrent Hepatocellular Carcinoma After Liver Transplantation: Analysis of Risk Factors. Transplant Proc 2016;48:2990-3. [Crossref] [PubMed]
- Zhu WJ, Huang CY, Li C, et al. Risk factors for early recurrence of HBV-related hepatocellular carcinoma meeting milan criteria after curative resection. Asian Pac J Cancer Prev 2013;14:7101-6. [Crossref] [PubMed]
- Zhou Y, Wang B, Wu J, et al. Association of preoperative EpCAM Circulating Tumor Cells and peripheral Treg cell levels with early recurrence of hepatocellular carcinoma following radical hepatic resection. BMC Cancer 2016;16:506. [Crossref] [PubMed]
- Lee TK, Castilho A, Cheung VC, et al. CD24(+) liver tumor-initiating cells drive self-renewal and tumor initiation through STAT3-mediated NANOG regulation. Cell Stem Cell 2011;9:50-63. [Crossref] [PubMed]
- Zhao RC, Zhou J, Chen KF, et al. The prognostic value of combination of CD90 and OCT4 for hepatocellular carcinoma after curative resection. Neoplasma 2016;63:288-98. [PubMed]
- Hong SW, Hur W, Choi JE, et al. Role of ADAM17 in invasion and migration of CD133-expressing liver cancer stem cells after irradiation. Oncotarget 2016;7:23482-97. [Crossref] [PubMed]
- He XX, Zhang YN, Yan JW, et al. CP-31398 inhibits the growth of p53-mutated liver cancer cells in vitro and in vivo. Tumour Biol 2016;37:807-15. [Crossref] [PubMed]
- Carr MI, Roderick JE, Gannon HS, et al. Mdm2 Phosphorylation Regulates Its Stability and Has Contrasting Effects on Oncogene and Radiation-Induced Tumorigenesis. Cell Rep 2016;16:2618-29. [Crossref] [PubMed]
- Yang Y, Xia T, Li N, et al. Combined effects of p53 and MDM2 polymorphisms on susceptibility and surgical prognosis in hepatitis B virus-related hepatocellular carcinoma. Protein Cell 2013;4:71-81. [Crossref] [PubMed]
- Chen M, Luo F, Yu J, et al. Common functional polymorphism within miR-146a and miR-196a-2 as susceptibility loci for hepatocellular carcinoma: An updated meta-analysis. Meta Gene 2015;7:40-7. [Crossref] [PubMed]
- Zhang Z, Zhang Y, Sun XX, et al. microRNA-146a inhibits cancer metastasis by downregulating VEGF through dual pathways in hepatocellular carcinoma. Mol Cancer 2015;14:5. [Crossref] [PubMed]
- Matsushima-Nishiwaki R, Toyoda H, Nagasawa T, et al. Phosphorylated Heat Shock Protein 20 (HSPB6) Regulates Transforming Growth Factor-alpha-Induced Migration and Invasion of Hepatocellular Carcinoma Cells. PLoS One 2016;11:e0151907. [Crossref] [PubMed]
- Lachenmayer A, Alsinet C, Savic R, et al. Wnt-pathway activation in two molecular classes of hepatocellular carcinoma and experimental modulation by sorafenib. Clin Cancer Res 2012;18:4997-5007. [Crossref] [PubMed]
- Mizuguchi Y, Takizawa T, Yoshida H, et al. Dysregulated miRNA in progression of hepatocellular carcinoma: A systematic review. Hepatol Res 2016;46:391-406. [Crossref] [PubMed]
- Huang YK, Fan XG, Qiu F, et al. Genomics of hepatitis B virus-related hepatocellular carcinoma and adjacent noncancerous tissues with cDNA microarray. Chin Med J (Engl) 2011;124:2057-64. [PubMed]
- Huang YK, Fan XG, Qiu F. TM4SF1 Promotes Proliferation, Invasion, and Metastasis in Human Liver Cancer Cells. Int J Mol Sci 2016;17:E661. [Crossref] [PubMed]
- Vianello F, Sambado L, Goss A, et al. Dabigatran antagonizes growth, cell-cycle progression, migration, and endothelial tube formation induced by thrombin in breast and glioblastoma cell lines. Cancer Med 2016;5:2886-98. [Crossref] [PubMed]
- Qu K, Wang Z, Fan H, et al. MCM7 promotes cancer progression through cyclin D1-dependent signaling and serves as a prognostic marker for patients with hepatocellular carcinoma. Cell Death Dis 2017;8:e2603. [Crossref] [PubMed]
- Tu K, Liu Z, Yao B, et al. BCL-3 promotes the tumor growth of hepatocellular carcinoma by regulating cell proliferation and the cell cycle through cyclin D1. Oncol Rep 2016;35:2382-90. [Crossref] [PubMed]
- Zhao Y, He HR, Wang MY, et al. Cyclin D1 G870A gene polymorphism and risk of leukemia and hepatocellular carcinoma: a meta-analysis. Genet Mol Res 2015;14:5171-80. [Crossref] [PubMed]
- Qie S, Diehl JA. Cyclin D1, cancer progression, and opportunities in cancer treatment. J Mol Med (Berl) 2016;94:1313-26. [Crossref] [PubMed]
- Yu H, Jiang HL, Xu D, et al. Transcription Factor MafB Promotes Hepatocellular Carcinoma Cell Proliferation through Up-Regulation of Cyclin D1. Cell Physiol Biochem 2016;39:700-8. [Crossref] [PubMed]
- Montalbano M, Curcuru G, Shirafkan A, et al. Modeling of Hepatocytes Proliferation Isolated from Proximal and Distal Zones from Human Hepatocellular Carcinoma Lesion. PLoS One 2016;11:e0153613. [Crossref] [PubMed]
- Yuan SX, Tao QF, Wang J, et al. Antisense long non-coding RNA PCNA-AS1 promotes tumor growth by regulating proliferating cell nuclear antigen in hepatocellular carcinoma. Cancer Lett 2014;349:87-94. [Crossref] [PubMed]
- Ma S, Yang J, Li J, et al. The clinical utility of the proliferating cell nuclear antigen expression in patients with hepatocellular carcinoma. Tumour Biol 2016;37:7405-12. [Crossref] [PubMed]
- Zafar H, Ali S. Boron inhibits the proliferating cell nuclear antigen index, molybdenum containing proteins and ameliorates oxidative stress in hepatocellular carcinoma. Arch Biochem Biophys 2013;529:66-74. [Crossref] [PubMed]
- Khalil MI, Ibrahim MM, El-Gaaly GA, et al. Trigonella foenum (Fenugreek) Induced Apoptosis in Hepatocellular Carcinoma Cell Line, HepG2, Mediated by Upregulation of p53 and Proliferating Cell Nuclear Antigen. Biomed Res Int 2015;2015:914645.
- Schneider JL, Cuervo AM. Liver autophagy: much more than just taking out the trash. Nat Rev Gastroenterol Hepatol 2014;11:187-200. [Crossref] [PubMed]
- Tai WT, Shiau CW, Chen HL, et al. Mcl-1-dependent activation of Beclin 1 mediates autophagic cell death induced by sorafenib and SC-59 in hepatocellular carcinoma cells. Cell Death Dis 2013;4:e485. [Crossref] [PubMed]
- Kowalik MA, Perra A, Ledda-Columbano GM, et al. Induction of autophagy promotes the growth of early preneoplastic rat liver nodules. Oncotarget 2016;7:5788-99. [Crossref] [PubMed]
- Zhu BS, Sun JL, Gong W, et al. Effects of 5fluorouracil and class III phosphoinositide 3kinase small interfering RNA combination therapy on SGC7901 human gastric cancer cells. Mol Med Rep 2015;11:1891-8. [Crossref] [PubMed]
- Lee YJ, Hah YJ, Kang YN, et al. The autophagy-related marker LC3 can predict prognosis in human hepatocellular carcinoma. PLoS One 2013;8:e81540. [Crossref] [PubMed]
- Tian Y, Wang L, Ou JH. Autophagy, a double-edged sword in hepatocarcinogenesis. Mol Cell Oncol 2015;2:e1004968. [Crossref] [PubMed]
- Wang SC, Lin XL, Li J, et al. MicroRNA-122 triggers mesenchymal-epithelial transition and suppresses hepatocellular carcinoma cell motility and invasion by targeting RhoA. PLoS One 2014;9:e101330. [Crossref] [PubMed]
- Hu M, Wang M, Lu H, et al. Loss of miR-1258 contributes to carcinogenesis and progression of liver cancer through targeting CDC28 protein kinase regulatory subunit 1B. Oncotarget 2016;7:43419-31. [Crossref] [PubMed]
- Qi X, Sun X, Xu J, et al. Associations between methylenetetrahydrofolate reductase polymorphisms and hepatocellular carcinoma risk in Chinese population. Tumour Biol 2014;35:1757-62. [Crossref] [PubMed]
- Hirata H, Sugimachi K, Komatsu H, et al. Decreased Expression of Fructose-1,6-bisphosphatase Associates with Glucose Metabolism and Tumor Progression in Hepatocellular Carcinoma. Cancer Res 2016;76:3265-76. [Crossref] [PubMed]
- Chung TW, Kim SJ, Choi HJ, et al. Hepatitis B virus X protein specially regulates the sialyl lewis a synthesis among glycosylation events for metastasis. Mol Cancer 2014;13:222. [Crossref] [PubMed]
- Zhu PP, Yuan SG, Liao Y, et al. High level of intercellular adhesion molecule-1 affects prognosis of patients with hepatocellular carcinoma. World J Gastroenterol 2015;21:7254-63. [Crossref] [PubMed]
- Saad A, Zhu XY, Herrmann S, et al. Adipose-derived mesenchymal stem cells from patients with atherosclerotic renovascular disease have increased DNA damage and reduced angiogenesis that can be modified by hypoxia. Stem Cell Res Ther 2016;7:128. [Crossref] [PubMed]
- Wang Y, Xing QF, Liu XQ, et al. MiR-122 targets VEGFC in bladder cancer to inhibit tumor growth and angiogenesis. Am J Transl Res 2016;8:3056-66. [PubMed]
- Zarei Mahmudabadi A, Masoomi Karimi M, Bahabadi M, et al. Inhibition of AGS Cancer Cell Proliferation following siRNA-Mediated Downregulation of VEGFR2. Cell J 2016;18:381-8. [PubMed]
- Tian J, Hu L, Li X, et al. MicroRNA-130b promotes lung cancer progression via PPARgamma/VEGF-A/BCL-2-mediated suppression of apoptosis. J Exp Clin Cancer Res 2016;35:105. [Crossref] [PubMed]
- Yoon C, Chang KK, Lee JH, et al. Multimodal targeting of tumor vasculature and cancer stem-like cells in sarcomas with VEGF-A inhibition, HIF-1alpha inhibition, and hypoxia-activated chemotherapy. Oncotarget 2016;7:42844-58. [Crossref] [PubMed]
- Meng W, Li X, Bai Z, et al. Silencing alpha-fetoprotein inhibits VEGF and MMP-2/9 production in human hepatocellular carcinoma cell. PLoS One 2014;9:e90660. [Crossref] [PubMed]
- Colombo J, Maciel JM, Ferreira LC, et al. Effects of melatonin on HIF-1alpha and VEGF expression and on the invasive properties of hepatocarcinoma cells. Oncol Lett 2016;12:231-7. [PubMed]
- Albini A. Extracellular Matrix Invasion in Metastases and Angiogenesis: Commentary on the Matrigel "Chemoinvasion Assay". Cancer Res 2016;76:4595-7. [Crossref] [PubMed]
- Roomi MW, Kalinovsky T, Niedzwiecki A, et al. Modulation of uPA, MMPs and their inhibitors by a novel nutrient mixture in human glioblastoma cell lines. Int J Oncol 2014;45:887-94. [Crossref] [PubMed]
- Wang X, Wang N, Li H, et al. Up-Regulation of PAI-1 and Down-Regulation of uPA Are Involved in Suppression of Invasiveness and Motility of Hepatocellular Carcinoma Cells by a Natural Compound Berberine. Int J Mol Sci 2016;17:577. [Crossref] [PubMed]
Cite this article as: Huang YK, Qiu F. Current perspectives of recurrence and progression in hepatocellular carcinoma. J Xiangya Med 2017;2:68.